Empirically validating the air change rate (ACH) via the direct measurement of aerosols
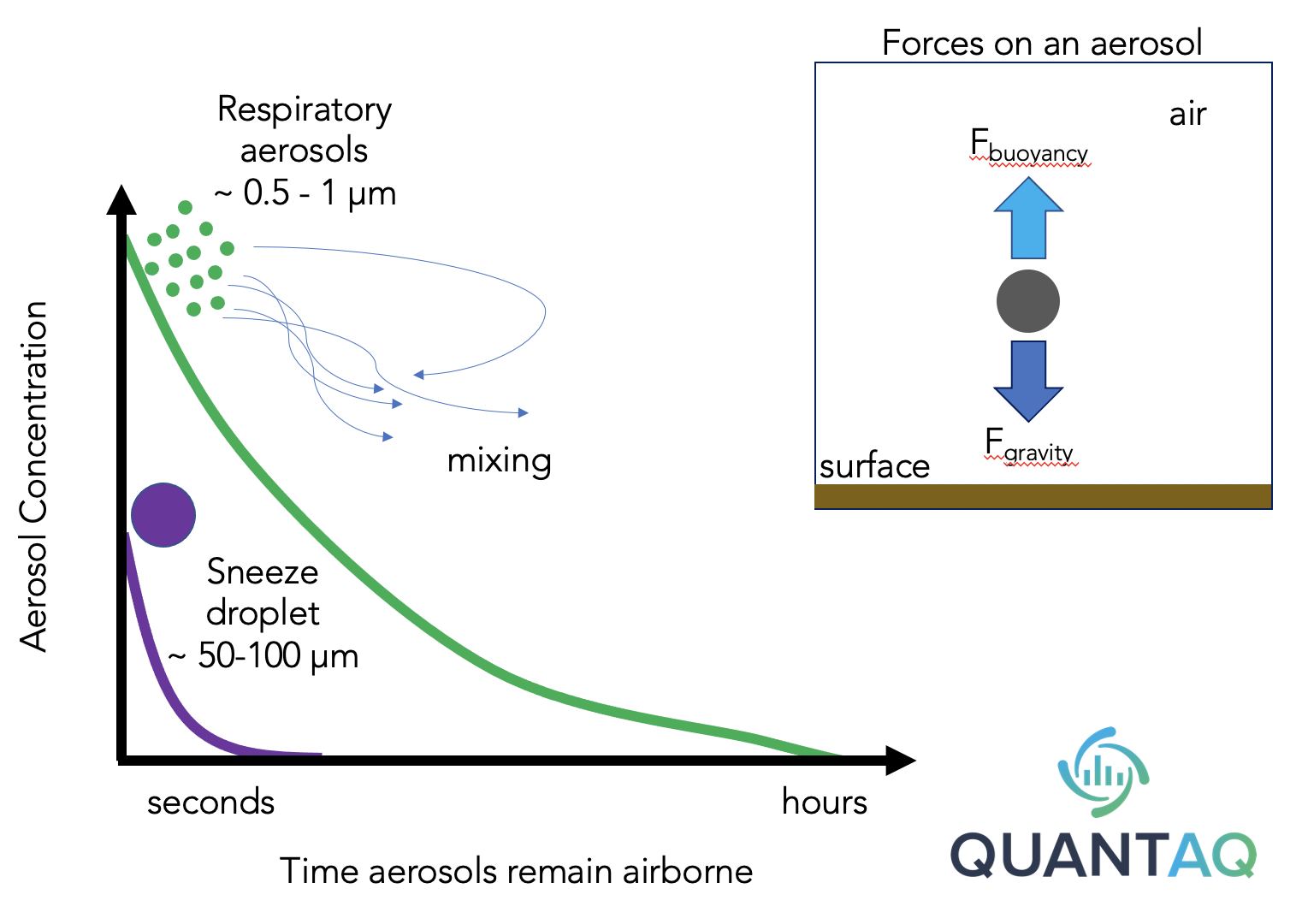
While aerosol awareness has grown substantially in 2020-2021 (thanks to COVID-19), the direct relationship between ventilation rates and indoor aerosol concentrations remains somewhat less clear. Here, we connect the dots between building ventilation and aerosols and demonstrate how low-cost particulate matter (PM) sensors can be used to empirically validate the air change rate in indoor environments.
Aerosols are tiny liquid or solid particles suspended in the air. The vast majority of aerosols are too small to be seen with the naked eye and persist in indoor (and outdoor) environments across a wide range of sizes, compositions, and residence times.
Respiratory aerosols are emitted as a byproduct of breathing, speaking, laughing, singing and are typically much smaller than those produced during a cough or a sneeze [Morawska, L. et al., 2009]. Because of their small size, respiratory aerosols can remain airborne for minutes to hours, allowing them to spread and linger within indoor environments. As a general rule, the larger the aerosol (i.e., the more it weighs), the faster gravity will force it to fall to the surface. Smaller aerosols experience a greater relative buoyancy force which keeps them airborne for longer periods of time.
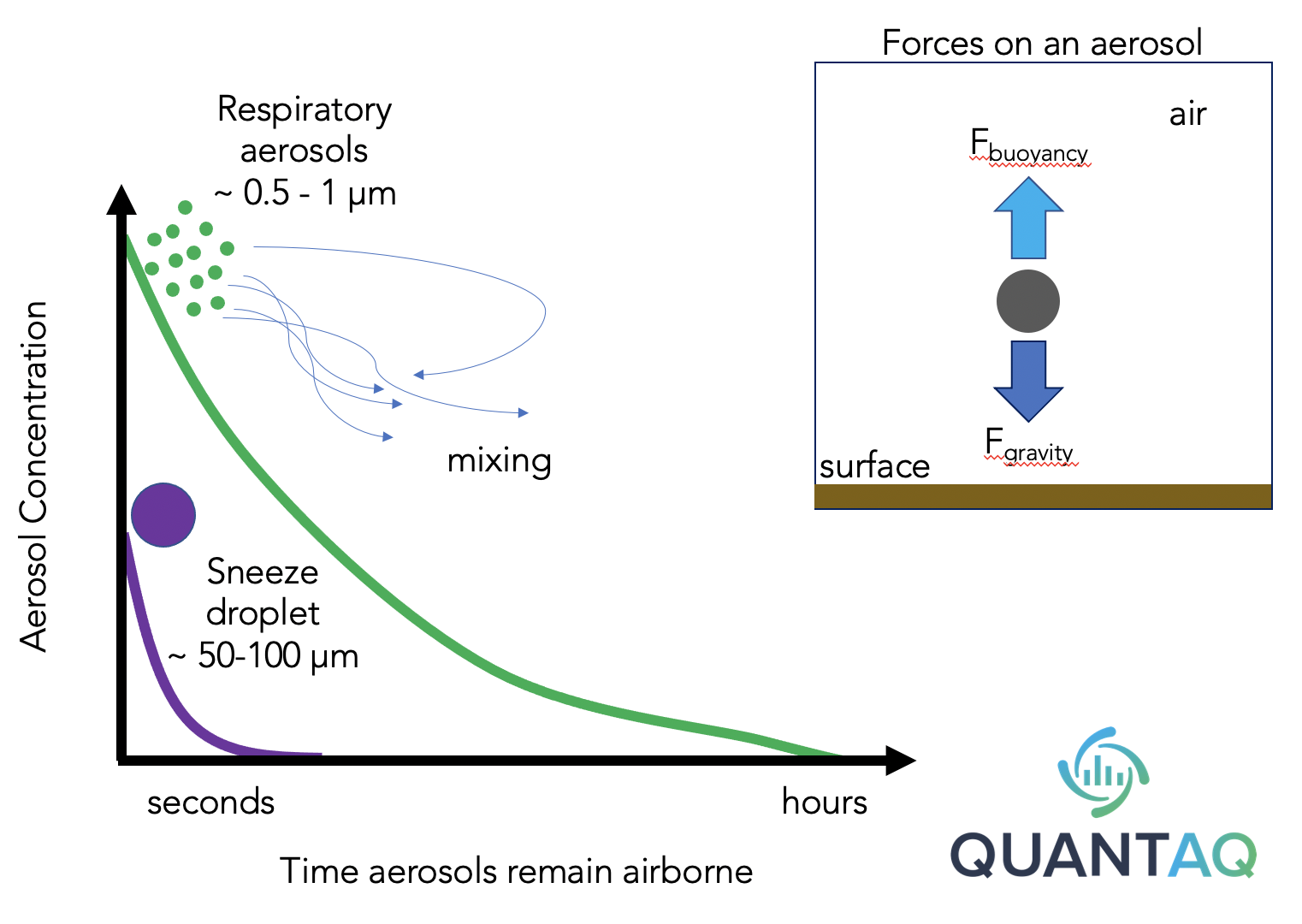
Can respiratory aerosols be measured in real-world environments?
Most indoor environments have elevated levels of aerosols from a variety of sources including cooking, cleaning, and/or moving around and kicking up dust. While it is possible to measure respiratory aerosols with relatively inexpensive particle sensors, it is practically challenging because the number of respiratory aerosols in the air is a small fraction of the total aerosol concentration. Non-respiratory aerosol sources tend to dominate real-world indoor environments. In this context, respiratory aerosols are the 'needles' and background aerosols are the 'haystack'. However, it is still possible to quantify the aerosol residence time (i.e., the time it takes the aerosols to be ventilated from a confined space) by making real-time measurements of aerosol concentrations. In other words, while we cannot necessarily measure the respiratory aerosols alone (i.e. the needles), we can measure the aerosol distribution that includes both the background and respiratory aerosol components (i.e., the haystack).
When working in indoor environments, we characterize the ventilation rate based on the number of Air Changes per Hour (ACH).
The ACH is the number of times per hour in which the total volume of air in a room is changed (or replaced) by an incoming flow of 'clean' air from the ventilation system.
This definition can be misleading if interpreted literally as air doesn't typically move through a room continuously as if it were toothpaste being pushed through a tube (i.e, in the real-world, air does not exhibit 'plug flow'). Instead, air mixes as it moves through indoor spaces. Take for example a 100 ft2 room with a 10 ft ceiling, the total volume of air in the room is 1000 ft3. If the ventilation system pushes 100 ft3 of air per minute (CFM), then the room receives 6,000 ft3 of 'clean' air every hour. The ACH is calculated as the volumetric flow rate of air (ft3/hr) entering the room divided by the volume of air in the room (ft3), so our ACH = 6 (6,000 ft3 hr-1/1,000 ft3 = 6 hr-1). A logical conclusion would then be that all the air in the room is 'exchanged' once every 10 minutes, or 6 times per hour. However, in reality, the air is effectively exchanged at a slower rate due to mixing. Because the air, and all the non droplet-sized aerosols suspended in the air, mix as they move around the room the time required to fully replace the 'older' air is longer than one might expect. The precise timescale over which the 'older' air is removed from the room is described using an exponential decay function. For a room with a known ACH, the 'older' air fraction remaining as a function of time can be calculated according to the equation:
$$'Older' Air Fraction Remaining = e^{-ACH*time} \tag{1}$$
where the ACH has units of hr-1 and time has units of hr. This is shown graphically in Figure 2. After 30 minutes, 95% of the 'older' room air has been exchanged with 'fresh' air from the ventilation system.
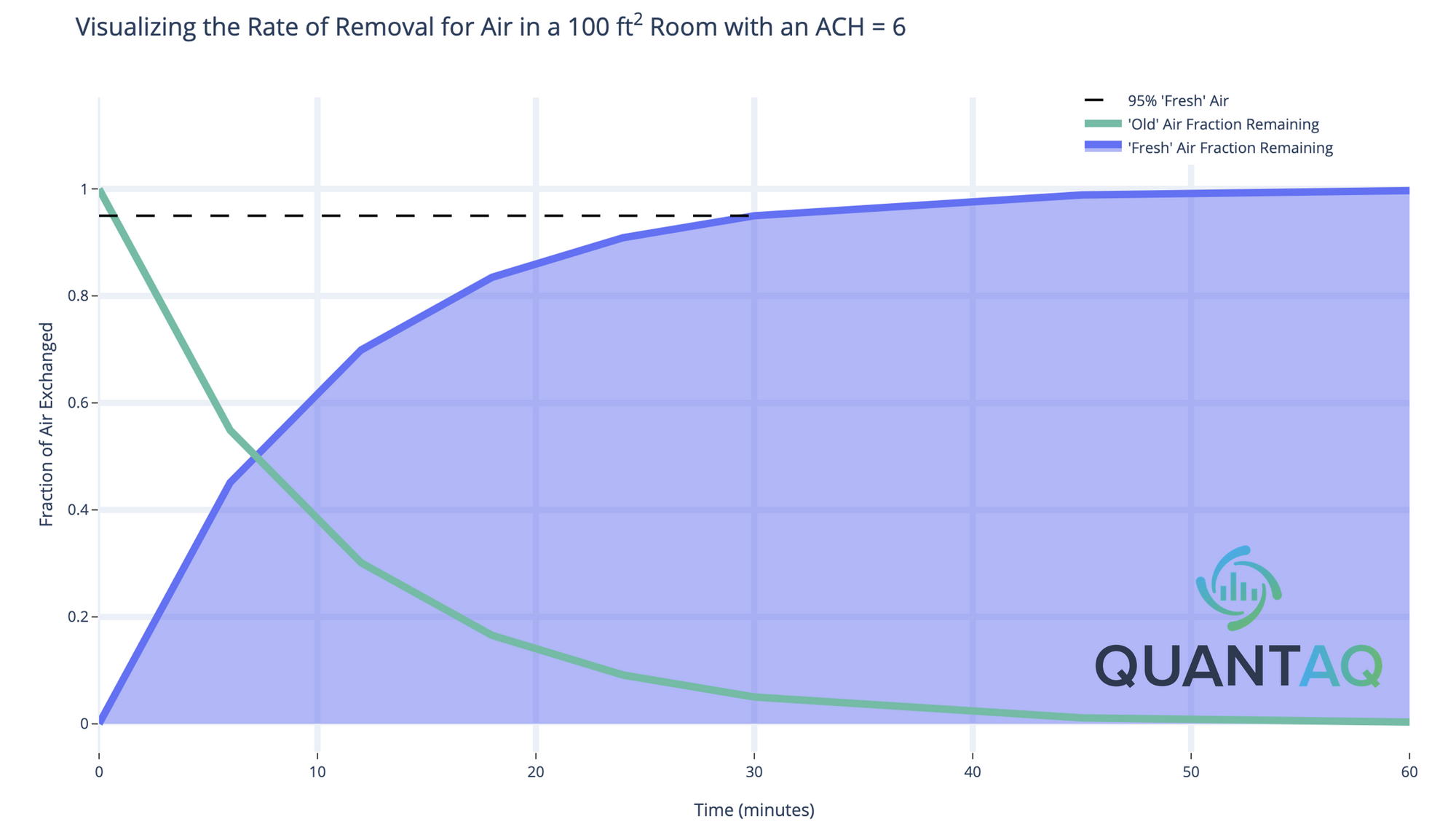
While it would be great if every room clearly stated its ACH on a big sign outside the door, many businesses, schools, restaurants, and workplaces are left with an incomplete understanding of their ventilation 'reality'. For rooms where the ACH is unknown, directly measuring the rate at which indoor aerosol concentrations decay using low-cost particle sensors allows one to empirically determine the ACH. Here, we demonstrate how you can use MODULAIR-PM (or other similar sensors) to verify the ACH of a room.
Experimental Details
In this experiment, we generate aerosols using an ultra-sonic nebulizer over the course of a 60-minute period, releasing the aerosols into a closed, actively ventilated ~240 ft2 room with 8 ft ceilings (V = 1,920 ft3 of air in the room). The room was equipped with three positive-pressure ventilation ducts in the ceiling, each pushing 100 ft3 per minute of 'fresh' air. One ventilation exhaust duct was also positioned in the ceiling, passively exhausting air from the room. Using the equation above, the ACH in this room should be 9.3 hr-1 and based on this ACH, it should take ~ 20 minutes to ventilate 95% of the aerosols from the room (after the aerosol generator is turned off).
To verify whether or not this is true, we used QuantAQ's MODULAIR-PM particulate matter sensor to obtain time-resolved measurements of the number, size, and mass concentration of the aerosols in the room. The sensor was placed in the center of the room at an elevation of ~3 ft. Figure 3 shows a time series of the number concentration of aerosols in the room. Following equation 1 above, we fit the data to an exponential decay curve (remember, real-world mixing) and report the value of tau (𝛕). Tau is the fit coefficient and tells us the time required to reduce the aerosol concentrations by 64% (or 1/e). We also report 3*𝛕 which corresponds with the timescale necessary to remove 95% of the aerosols.
Using this data, we can directly calculate the room's ACH (deemed 'aerosol-measured ACH') under each of the following test conditions and compare the results to the ventilation-based ACH.
Test Condition 1: In-room HEPA filter ON or OFF
Test Condition 2: Door to room left OPEN or CLOSED
Hypothesis
The ventilation-based ACH of 9.3 (based on business-as-usual (BAU) building operations) will most closely match the aerosol-measured ACH with no HEPA filter running in the room and the door CLOSED. With the door left OPEN, we expect the aerosol-measured ACH to be greater than the ventilation-based ACH. With the HEPA filter running in the room, we expect the aerosol-measured ACH to be much greater than the ventilation-based ACH.
Results
Below, we show the aerosol number concentration as a function of time for aerosols with diameters between 0.35 µm and 0.46 µm.
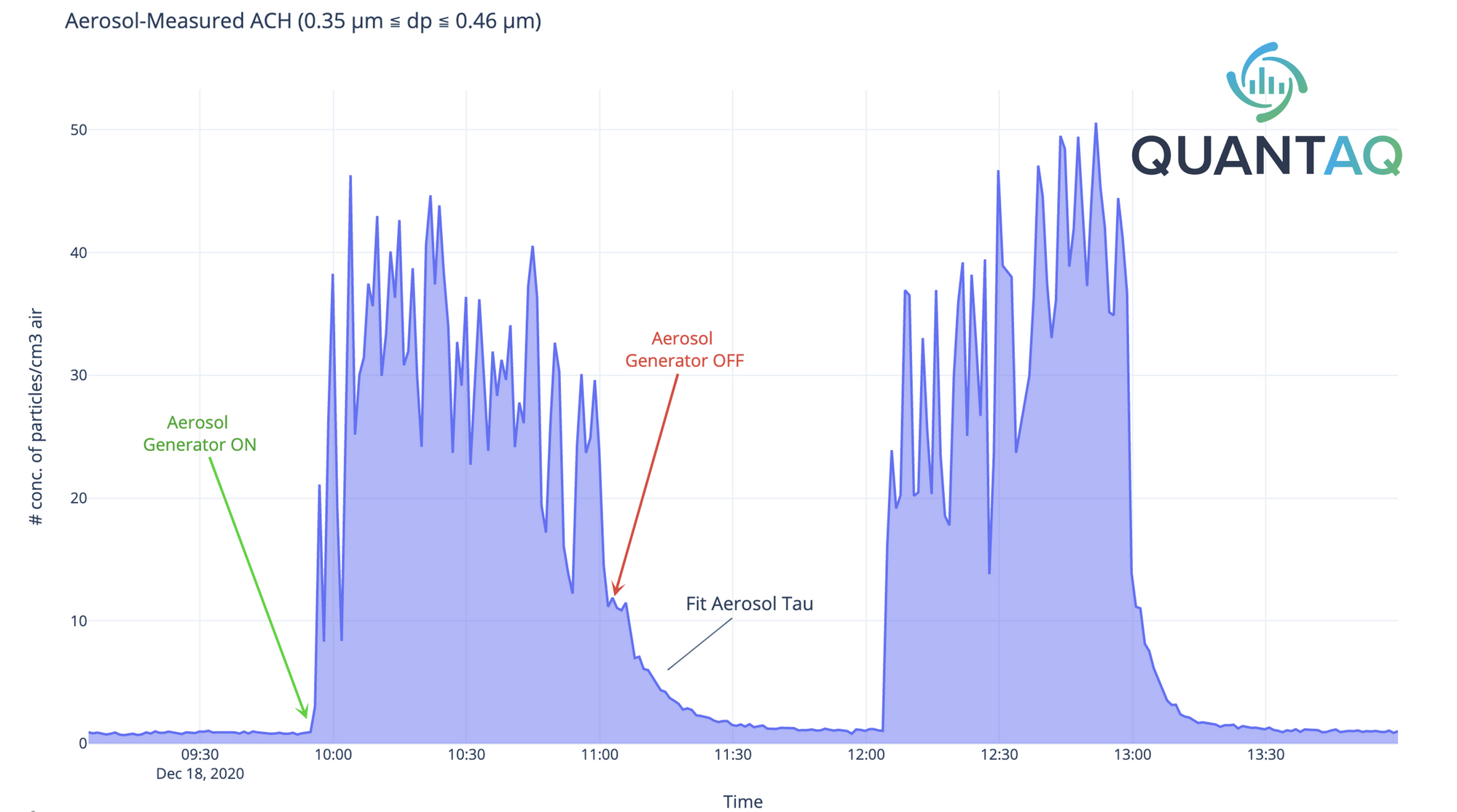
At 10 AM, the aerosol generator is turned ON and left on for an hour, increasing the aerosol concentrations in the room. At 11 AM, the aerosol generator is turned OFF and the aerosol levels decay. In this experimental run we characterized the decay rate (captured after 11 AM) with the in-room HEPA filter turned ON and the door left CLOSED. The same process is repeated between 12 and 1 PM, this time with the in-room HEPA filter turned ON and the door OPEN.
The results are presented in the table below for all four experimental runs.
Experiment Run No. | HEPA Filter State | Door State | 𝛕 (min) | 95% CI (min) | ACH (aerosol-measured) |
---|---|---|---|---|---|
1 | ON | OPEN | 4.5 | 13.5 | 13.3 |
2 | ON | CLOSED | 6.9 | 20.7 | 8.7 |
3 | OFF | OPEN | 9.1 | 27.3 | 6.6 |
4 | OFF | CLOSED | 13.4 | 40.2 | 4.5 |
Take homes
- With the door CLOSED and in-room HEPA filter turned OFF the aerosol-measured ACH was 4.5, 52% lower than the ventilation-based ACH.
- With the door OPEN and in-room HEPA filter turned OFF, the aerosol-measured ACH was 6.6, 29% lower than the ventilation-based ACH value.
- Adding an in-room HEPA filter to the room increased the aerosol-measured ACH by nearly 2x in both door CLOSED and OPEN conditions, resulting in aerosol-measured ACH values of 8.7 and 13.3 respectively.
- The single passive exhaust duct appears to substantially slow down the 'actual' ACH, limiting the rate at which air can escape from the room.
- For classrooms, conference rooms, and other indoor environments that rely on an assumed leak-rate of air from the room, it is important to not only consider how much air is being added (via the ventilation system or open windows), but also to understand how easily that same air is able to leave the room, especially under normal everyday use patterns.